The need to integrate a circular economy approach into the renewable energy transition is clear. While there are barriers in the current system to overcome, a number of pioneering businesses and policymakers are forging ahead to create solutions. Their efforts, especially if bolstered by others, can lead to a renewable energy sector that not only produces cleaner energy but is delivered via infrastructure that, by design, eliminates waste and pollution, keeps the materials it needs in use, and regenerates nature. This model, a circular economy, will lead to greater benefits for the environment, people, and business.
It's time for a redesign. Solar panels and wind turbine blades ending up in landfill are flaws in product and business model design. In the same way, allowing materials to become waste after use while virgin materialsvirgin materialsMaterials that have not yet been used in the economy. are extracted to make new renewable energyrenewable energyEnergy derived from resources that are not depleted on timescales relevant to the economy, i.e. not geological timescales. technologies is a system design flaw.
Adopting a circular economycircular economyA systems solution framework that tackles global challenges like climate change, biodiversity loss, waste, and pollution. It is based on three principles, driven by design: eliminate waste and pollution, circulate products and materials (at their highest value), and regenerate nature. approach to the development of the renewable energy sector offers solutions to these shortcomings. Indeed, interest in how a circular economy approach can help tackle the 45% of greenhouse gas (GHG) emissions from how we make products and manage the land is beginning to take its place as part of the global climate agenda. It is central to the vision of the US Department for Energy’s National Renewable Energy Laboratory, and has been highlighted as underpinning “the “sustainability credentials” of Europe’s renewable energy transition by the European Environment Agency (EEA). It is also prominent in China’s 14th Five-Year Plan in relation to batteries as well as the metals needed for solar panels and wind turbines.
Integrating a circular economy approach into renewable energy infrastructure development – securing resources and tackling materials-related environmental impacts – requires a redesign of technologies, business models, and policies. It offers the opportunity to create a better system, in which waste and pollution are eliminated, material consumption is reduced, products and materials are circulated, and space is left for nature to thrive.
So where to begin?
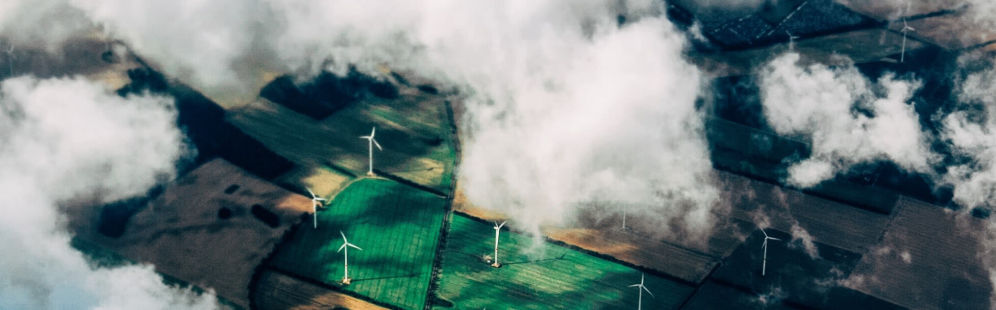
Redesigning use cycles: lifetime extension
One clear circular economy approach is to extend the lifetimes of existing technologies and in turn significantly reduce material needs. As a general rule, the expected lifetime of both wind and solar technology is around 25 years. Extending the life of a 500 megawatt wind farm by 10 years could over the course of a century reduce demand for copper alone by around 4,400 tonnes, while reducing the greenhouse gas (GHG) emissions and impacts on biodiversity associated with copper mining and production.
Perhaps the simplest example of lifetime extension is regular maintenance. In wind farms, keeping blades and components clean and in good working order reduces downtime and costs. Service concepts have become increasingly sophisticated as technology has developed, with providers and consortiums, such as Siemens and BladeSave, offering condition monitoring service systems which use a series of sensors to detect anomalies, vibrations, and weather conditions, and advise corrective actions early.
In the solar sector, an economic model has been proposed that focuses on maintenance at regular intervals. If applied, the model predicts that modules installed today should be viable for 50 years before they need to be replaced.
Repairing and updating equipment is also impactful. The fastest moving parts in a turbine are subject to the greatest wear and tear, namely the blades and the gearbox. Leading edge erosion is common in turbine blades, for example, but polymer pastes, vacuum assisted crack injections, and patch repairs have been developed to mend – in situ – cracks, pitting, delamination, and other problems. Retrofitting individual blade controls can minimise the strain on them, making the parts last longer.
When they do eventually wear out, many parts can be remanufactured to prolong the overall life of the asset. Components such as gearboxes, generators, bearings, and rotors that are predominantly made of steel can be readily remanufactured to return them to their original specification. Remanufacturing is an ‘inner loop’ of the circular economy as it maintains more of the embedded energy and labour that went into making the original components compared to ‘outer loop’ strategies like recycling.
In a lifetime extension scenario, worn components are replaced while keeping the towers, foundations, and electrical grid installation in place. Installing refurbished blades and nacelles in five offshore wind turbines at Bockstigen in Sweden after 20 years in service increased their capacity from 2.75 megawatts to 3.3 megawatts and extended their productivity by 15 years.
The opportunities of maintaining and refurbishing renewable energy assets are increasingly being taken up by pioneering organisations. Renewable Parts and the University of Strathclyde are exploring opportunities to remanufactureremanufactureRe-engineer products and components to as-new condition with the same, or improved, level of performance as a newly manufactured one. and refurbishrefurbishReturn a product to good working order. This can include repairing or replacing components, updating specifications, and improving cosmetic appearance. components, Siemens Gamesa has a Life Extension programme that looks to add 10 years to the lifetime of a turbine, and Spectrum Energy Services offers a dedicated supply and install service for remanufactured turbines.
Recognising the benefits of lifetime extension, businesses are beginning to redesign renewable technologies to be easy to maintainmaintainKeep a product in its existing state of quality, functionally and/or cosmetically, to guard against failure or decline. It is a practice that retains the highest value of a product by extending its use period. and repairrepairOperation by which a faulty or broken product or component is returned back to a usable state to fulfil its intended use. from the outset. Energy company Enel, for example, highlights repair and lifetime extension as part of its circular design strategy with enablers including design for disassembly, standardisation, and modularity. Turbine manufacturer Vestas has put modularity at the forefront of its latest nacelle design, with the prototype released in August 2022 forming “a solid foundation for future products and upgrades,” according to Chief Technology Officer Anders Nielsen.
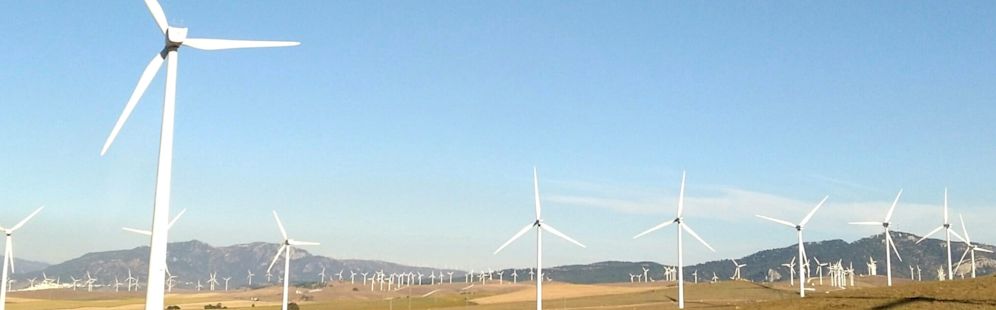
Redesigning use cycles: repowering
Upstream design strategies are crucial to long-term material availability. While lifetime extension keeps materials in use and lessens environmental impacts, leaving space for nature to thrive, in certain circumstances there is a strong argument for full repowering – entirely replacing the technology.
Existing wind farms are often located in the best wind positions, which means replacing turbines with the latest technology can maximise the location’s natural resource. Modernising a wind fleet can require fewer turbines to generate greater electricity output. Fewer turbines can mean fewer materials overall. Popular examples include the Windplan Groen project in the Netherlands where 98 turbines are being replaced by 90 – while increasing capacity from 168 megawatts to 500 megawatts – and Hagshaw Hill Windfarm in the UK, where 26 turbines will be replaced with 14 larger and more efficient turbines, delivering six times the amount of electricity.
However, it isn’t always the case that the quantity of materials used decreases with bigger but fewer turbines. Anne Velenturf, Senior Research Fellow in Circular Economy at the University of Leeds, notes that there is analysis to support both conclusions. There is no categorical answer.
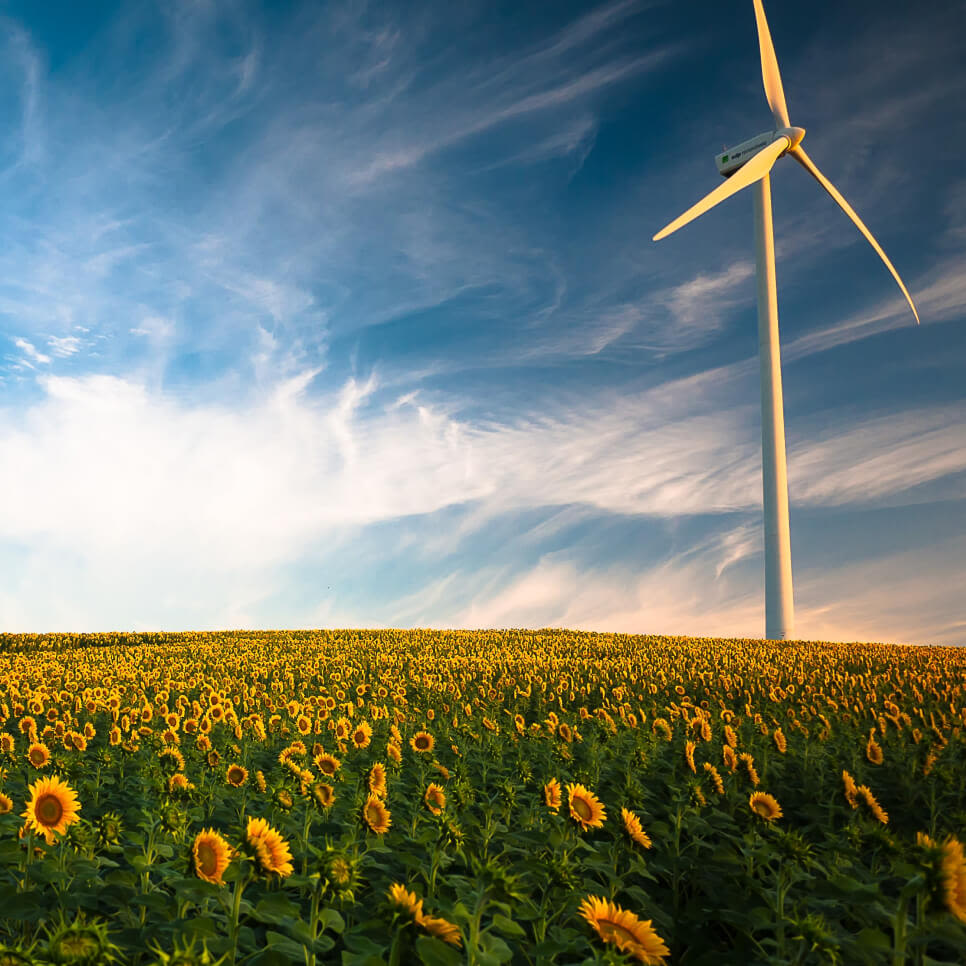
Repowering wind farm locations can avoid additional land use change, a key driver of biodiversity loss. In fact some academics are exploring the development of biodiversity enhancement measures on wind farms, treating them as mini wildlife reserves. The EU Commission issued a notice on how to “reconcile wind energy developments with nature” onshore and offshore, highlighting instances where repowering could be beneficial to local biodiversity in comparison to maintenance of existing infrastructure. A model of collision risks focused on the white-tailed eagle population in Norway found repowering with larger turbines would reduce the collision risk by more than two thirds compared to the existing wind farm.
Though it has significant material implications, repowering can be very impactful when it comes to increasing energy generation – reducing reliance on fossil-fuels, and therefore decreasing their consumption and related emissions. Given that such an approach does mean existing infrastructure is replaced, this in fact makes the case for ensuring all infrastructure is designed for future use possibilities even stronger.
No matter the renewable energy technology in question, the solution is to get the use cycles right. Wind farms that exist today, if they are effective, need to be maintained, refurbished, and repaired in order to maximise environmental and other benefits. But if a wind farm could be more effective were it repowered then there need to be considerations around where the materials to build the new infrastructure come from and where materials from the old infrastructure go so that they keep adding value in the economy.
That requires technology providers to source materials from within the industry and the economy more broadly, rather than turning to extraction of finite materialsfinite materialsMaterials that are non-renewable on timescales relevant to the economy, i.e. not geological timescales.. It requires decommissioning guidance and financing to be made available to ensure products and components are reused or, if that isn’t possible, their materials recycled (either within the renewable energy industry or in other industries), and designing for these purposes. Policy is needed to support reusereuseThe repeated use of a product or component for its intended purpose without significant modification. when it is appropriate and, when it isn’t, to support systems that keep the materials in the economy and out of landfill.
Crucially, all possible use cycles should be planned for at the design stage. As Radjou and Prabhu in their book Frugal Innovation argue, “over 70% of a product’s life-cycle costs and environmental footprint is determined during its design phase,” and once decisions are implemented, it’s hard to reverse their impacts.
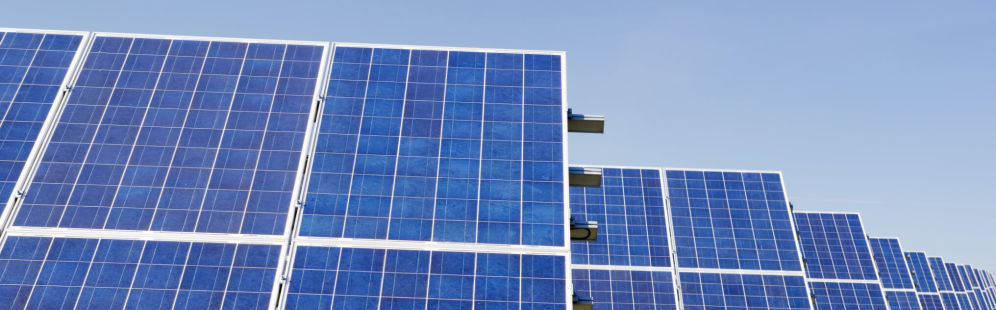
Designing for reuse
“The transition is already happening. And it's happened despite a lot of headwinds. So that's encouraging.”
Parikhit Sinha, Director of Sustainable Development, First Solar
Designing for reuse involves both system and product design.
Take a solar array (a collection of solar panels), for example. Due to its size and structure, generally, a solar array is easy to reuse in its original form when simply moved elsewhere. But for this to happen in practice, systems need to be created to enable reuse.
Nascent secondary markets for solar technology are already being developed. Though hard data on these markets is lacking, the secondary market for photovoltaic (PV) cells in the US has been described as “showing promising growth and traction”; industry insiders say the pan-European secondary market is “very healthy”; and research in Japan has shown a rising trend for used solar PV equipment.
In addition to traditional marketplaces, new models for reuse are emerging. Since 2018, the European Commission funded project Circusol – established to explore ‘sustainable business models’ for the renewable energy sector – has piloted several product service systems (PSS) for solar and battery technologies that encourage reuse.
In Circusol’s model, solar panels and batteries remain the responsibility of the supplier after they have been installed for use. This means the supplier is both responsible for ensuring the technology functions well over time and also for deciding when it has come to the end of its usable life. If a solar panel or battery becomes unsuitable for use at its first location, the supplier can take it back and assess whether it can be used somewhere else.
This model encourages product design to be focused on durability, reuse, repair, refurbishment, and remanufacturing, as each strategy can benefit the supplier’s bottom line.
Reuse strategies look slightly different in the wind sector. The sheer size of the technology means few wind turbines are transported (in their large component parts; blades, tower etc), to new locations for reuse – although a handful of brokers, like the Netherlands’ Dutchwind, match buyers with second-hand turbines to resite, and there are other noted instances of reuse of refurbished turbines. Structural reuse though, where a product is segmented into its construction elements and they are reused, is more common.
Modularity and design for disassembly are vital to structural reuse. Vestas’ latest developments in modular ‘click-on’ nacelle design, for example, not only aim to make transportation, customisation and upgrades easier, but also allow for reuse in future. Lattice-style towers both use less steel and are easier to disassemble and use again. Innovators are even working to develop modular blades, and modular, multi-turbine micro-wind solutions.
Structural reuse can be economically, as well as environmentally, appealing. James Barry notes that his business Renewable Parts, which works with the steel components of wind turbines, can, in some cases, provide parts at a much lower cost – up to a 40% reduction – compared to a new part because the business is not fabricating from fresh. And, because the business tracks the component journey and tests before resale, it will always put a full warranty around the parts as if they were new.
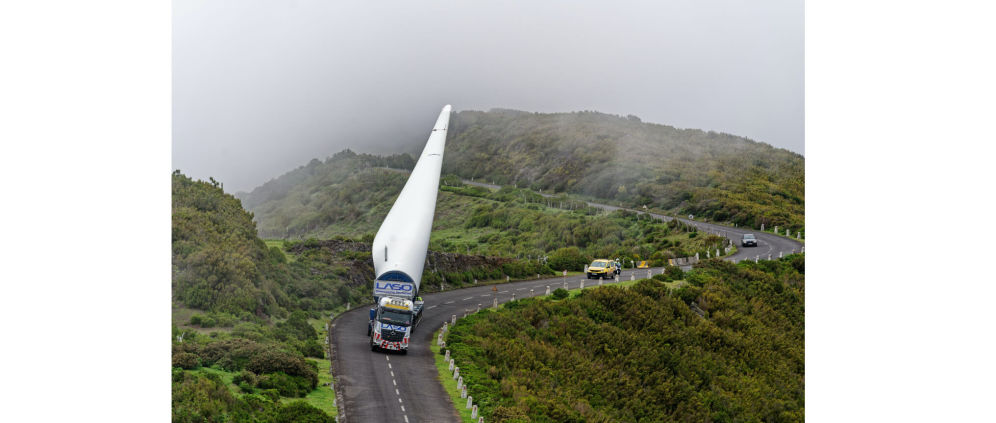
Despite this economic rationale, Barry says that it is only in recent years that refurbished parts have really gained traction in the wind industry. “The idea, in 2011, was ahead of its time. You couldn't get anyone in the wind industry back then interested in this idea that they should be buying refurbished parts. But in the last two to three years, people have become much more engaged and interested in solutions.”
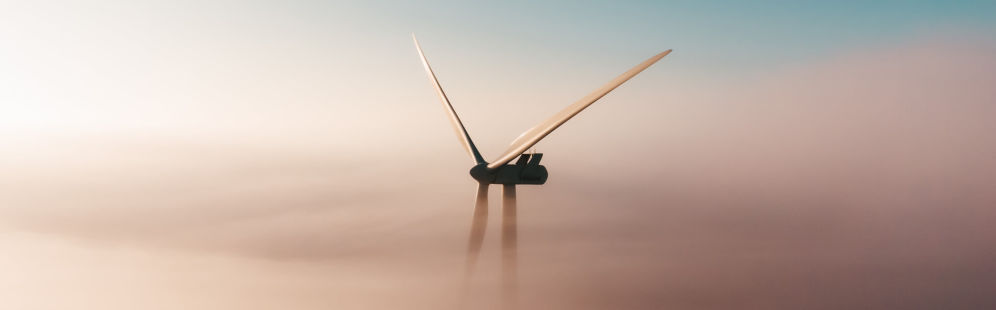
Designing for recycling
While designs of products and systems for reuse seek to maintain the integrity of an asset to the greatest extent possible, designing for recycling is equally necessary so that, as technologies evolve and are redesigned, their materials can be kept in the economy and don’t become waste.
Present designs of renewable technologies can make recycling challenging. For example, while up to 85% of a wind turbine can, in principle, already be recycled – including the mast and electrical components for which there are established recycling processes – the hard-to-manage composite turbine blades pose the biggest challenge, and currently make up 10% of Europe’s total fibre-reinforced composite waste.
As a first port of call, organisations such as ReBlade and Enel are developing after-use value chains for decommissioned blades, channelling the composites into products such as bridges and electricity poles. In 2022 Enel set out to build a new recycling centre in Spain for this purpose. These are necessary solutions to the growing waste problem. But the use of turbine blade waste in other sectors, in many cases, may only be delaying its journey to landfill rather than halting it indefinitely.
Investment in processes that separate the fibres, resin, and chemicals in the blades so that they can be used in the manufacture of new blades is therefore needed. Both Enel and ReBlade are investigating potential fibre reclamation technologies, with plans to invest. Meanwhile, Vestas has launched a technology that should enable industrial-scale recycling of turbine blades by 2024.
Crucially, Vestas has also committed to designing blades that can be 100% recycled by 2030, in an effort to avoid downcycling of blade materials. The company’s approach highlights the need to not only address blade waste at end-of-use but to design it out in the first place.
For all materials to be circulated, recycling needs to be considered at the design and planning stages of technology development – not only to eliminate waste but to secure secondary material supplies for future designs, thereby improving the sector’s resilience to supply chain shocks.
In the solar sector, PV designs mean recycling is often focused on bulk materials such as glass and aluminium. Circulation of these materials is important but equally crucial is the recovery of high-value semiconductor used in smaller quantities. This includes silicon and critical raw materials (CRMs) such as indium and cadmium, which the Royal Society of Chemistry notes are often sacrificed “in favour of higher value materials,” during the commonly-used pyrometallurgical recycling process.
Recycling processes to effectively recover semiconductors from PV cells do already exist. In Germany, a new system to recycle silicon from PV cells has been developed – with crystalline silicon cells presently the dominant solar technology – and the EU-funded project PHOTORAMA will pilot similar technologies to disassemble, separate, and recover metals from solar cells by 2024.
Like in the wind industry though, solar technologies need to be designed to make recyling effective and easy. First Solar, which manufactures Cadmium Telluride (CdTe) thin film solar cells, has for the past 15 years operated global in-house PV recycling capabilities that can recover up to 95% of semiconductor materials due to their design.
Among the strategies recommended for PV panel design by the International Energy Agency (IEA) are: minimising the use of hazardous and hard-to-recover materials, particularly in the metallic backsheet; and choosing reversible adhesive bonds, encapsulants and sealants to aid disassembly. Industry collaboration to standardise panel design is another important strand of circular design, alongside the introduction of material passports to track and disclose material origin and composition. The EPEAT ecolabel includes criterion on material recovery targets and requires material composition datasheets to be made available to recyclers.
At the moment, only a handful of companies consider end-of-use at the design stage. While there are clear benefits to be gained from more businesses adopting this approach, Scott Bryant, Circular Economy Coordinator for the City of Greater Bendigo, and former Circular Energy Infrastructure Lead at Zero Waste Scotland, notes that “this needs to be carefully balanced” as there can be unintended consequences of design changes. He points to the reduction of cobalt content in lithium-ion batteries as an example of where design changes for good reason – in this case, to bring down battery costs – resulted in the reduced value of old batteries to recyclers, impacting “the viability of the whole lithium-ion battery recycling process.”
While caution should be exercised, however, shifting the focus upstream, to the design stage, could be transformative for the renewable energy sector.
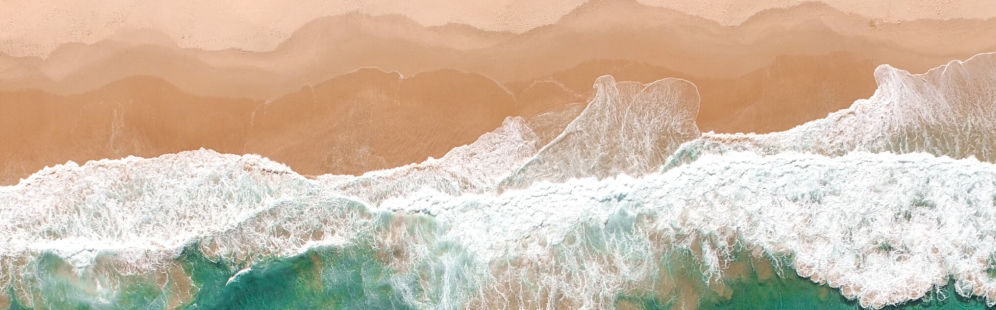
Rethinking the economics
Alongside the array of design considerations that go into building renewable energy infrastructure using circular economy strategies, there are also economic levers to think about.
In the solar sector, for example, the economics of recycling can encourage waste over materials circulation. According to the National Renewable Energy Laboratory (NREL) in 2020, in the US it cost an estimated USD 20-30 to recycle one PV panel, while sending that same panel to landfill cost as little as USD 1-2. And yet, a 2016 report from IRENA and the International Energy Agency Photovoltaic Power Systems Programme (IEA-PVPS) highlighted that recycling or repurposing solar PV panels at the end of their use, if the materials are “fully injected back into the economy”, could represent an opportunity of more than USD 15 billion by 2050.
The decision whether a renewable energy technology continues to be used is often based on the levelized cost of electricity (LCOE), a calculation which takes into account not only the energy that can be generated but expenditures such as operation and maintenance costs across an asset’s use. Parikhit Sinha, Director of Sustainable Development at First Solar, explains that “it comes down to the economics. Are you meeting your targets for rate of return? Do you still have a contract with a customer to buy that power that justifies keeping the plant running? And is there a new technology that's low cost and higher efficiency that means you should replace them?”
While after 25 years a solar panel is still able to absorb around 80% of the energy it can at its peak, current LCOE calculations can encourage shorter lifetimes for solar installations. This would change, however, if the cost of solar panel waste was factored into these calculations, landing the responsibility for their disposal with businesses. A 2021 article in Harvard Business Review noted that by as soon as 2035, discarded panels could outweigh new units sold by 2.56 times and that “this would catapult the LCOE [...] to four times the current projection.” The economics of solar, described as “so bright-seeming” at present, would “darken quickly as the industry sinks under the weight of its own trash.”
Such projections support the need for circular economy solutions, encouraging new research and experimentation to keep technologies in use for longer and stimulating supportive policies and incentives. In 2021, the IEA-PVPS assessed the environmental and financial viability of circular economy scenarios, finding that it is not only better for the environment to keep a solar panel in use instead of replacing it with new panels but that it also “proves financially competitive” in comparison to the “recyclerecycleTransform a product or component into its basic materials or substances and reprocessing them into new materials. and acquire new” scenario from a theoretical LCOE perspective.
There are some conditions for this. The study concludes that, in practice, “the potential to satisfy the lifetime of a prematurely decommissioned PV panel through repair or direct reuse is often considered financially unattractive” and that this option is currently therefore considered “niche”. It also adds that reuse requires “efficient rehabilitation and repair processes, recertification procedures for prematurely decommissioned PV, and transparent communication about the remaining lifetime and peak power of prematurely decommissioned panels." This doesn’t detract from the promise of reuse as much as it reinforces the need for the system conditions to be right for it to work. Here, there is a significant role for policy to play.
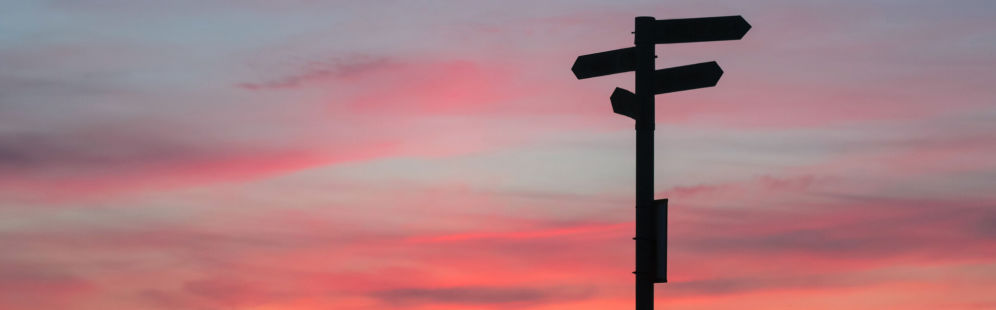
Rethinking policies
“It seems we didn't really start thinking about this early enough. And now we're in a situation where we desperately need policy and consideration given to these issues”
Rebecca Windemer, ESRC Postdoctoral Fellow, Cardiff University
Policymakers can play a key role in enabling the economics to work. For example, the European Union (EU) Waste Electrical and Electronic Equipment (WEEE) directive mandates that the cost of collection, sorting, and reuse or recycling of technologies, which includes solar panels, be borne by ‘producers’.
However, there is a need for further development, implementation, and policing of regulations such as this. “With Extended Producer Responsibility, theoretically, all PV panels should be recycled, at least in Europe,” explains Tom Rommens, coordinator of Circusol. “But what we see in practice is that these panels are diffused in the markets and end users are not always aware of what to do with them. Many of them are recycled, but a lot of them are lost somewhere in second life circuits [...], used for a couple of years, and then they end up on a waste pile somewhere else. That's a situation that we can no longer accept.”
In addition to this challenge, Sinha explains that even when technology is successfully collected and sorted for recycling, businesses in Europe “can still meet the WEEE directive recovery targets of 85% by focusing only on the bulk materials” of the solar panels, which make up the majority of their weight, rather than the precious metals and semiconductor materials, which typically make up less than 15%.
The European Committee for Electrotechnical Standardisation (CENELEC) has developed a supplementary standard, specific to PV panel collection and treatment, to assist recyclers with recovery of the semiconductor materials. But, as this standard is voluntary there is still room for much-needed development in this area.
Beyond collection and recycling, policy development is also needed to support further adoption of reuse opportunities. Looking to the EU again, the region’s Waste Framework Directive currently defines reuse of an item as “any operation by which products or components that are not waste are used again for the same purpose for which they were conceived.” This is problematic if technologies move between different end uses: it is already preventing reuse of EV batteries for utilities and off-grid storage as this is considered downcycling and not permitted.
In 2021, a study conducted by Belgian research institute collective EnergyVille and non-profit PV CYCLE concluded that legislation in the EU related to solar technology reuse is currently unclear and that technical guidelines and standards are needed, outlining the sorting and testing steps required to qualify modules for reuse.
"We'd like to have at least a European, or an international, standard describing how to test [the technologies] and giving some guidance on what kind of defects, for example, are acceptable with refurbishment, and which types of defects are no longer acceptable. What would make a panel ready to be recycled and not reused anymore.”
- Tom Rommens, Coordinator, CIRCUSOL
Policy barriers have also been noted in the wind industry. A Spanish study found that the biggest barriers to refurbishing existing wind turbine stock are regulatory and administrative, outweighing any economic, environmental, and technical considerations. In the EU, only Italy offers a concrete incentive for lifetime extension projects for wind farms.
Other regulatory areas also lag behind the latest technological developments. In planning policy, for example, most licences to build renewable energy infrastructure in the UK stipulate a 25-year arrangement, something which Rebecca Windemer, ESRC Postdoctoral Fellow at Cardiff University, highlights as being an “anomaly” when compared with consents for other infrastructure developments. The 25-year consents are, according to Windemer’s research, a legacy from the early days of renewable energy infrastructure.
But there is some good news. Such anomalies have started to be recognised in regions now leading the transition to renewable energy, such as Scotland, which has published policies stating sites should be suitable for use in perpetuity. And Deloitte, noted that “regulators and policymakers have started exploring solutions for extending the life and increasing the performance, recovery, and reuse” of renewable energy infrastructure, identifying end-of-use strategies as one of five renewable energy trends to watch in 2022. Progress is being made.
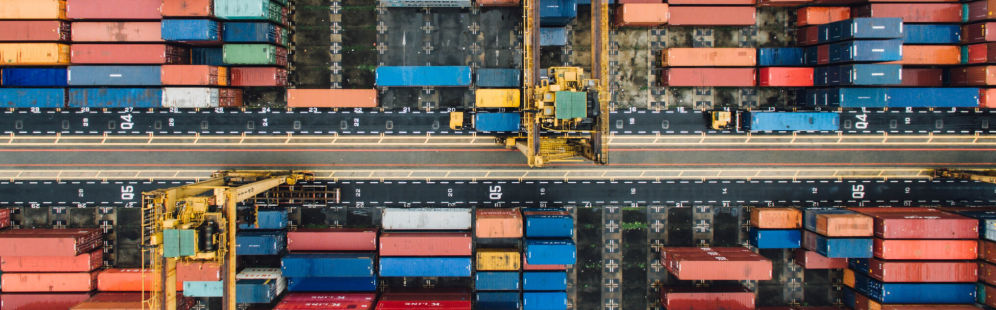
Actions of other industries can make a difference
While the renewable energy industry and policymakers have the biggest role to play in enabling a circular economy for this infrastructure, there is an important role for other industries to play: energy needs are inherently tied to all other economic activities.
Although we know we need to build lots of renewable energy infrastructure now as part of the energy transition away from fossil fuels, we’ll need increasingly more renewable energy infrastructure because of rising demand for electricity in other systems of the economy.
Take fashion for example. Around 80% of the energy used in the fashion industry is consumed by textile manufacturing. Many textile manufacturers have therefore sought innovative ways to increase energy efficiency. Such measures play an important role in reducing greenhouse gas emissions within the current system. More energy efficient processes should reduce demand for energy and so this is also an important part of the energy transition. But there is a limit to the impact energy efficiency can have on reducing energy needs.
Under the linear economylinear economyAn economy in which finite resources are extracted to make products that are used - generally not to their full potential - and then thrown away ('take-make-waste')., as textile manufacturing has become more energy efficient, production costs have decreased and production volumes have increased. From 2000 to 2015, clothing production doubled while, over the same period, utilisation – the number of times an item of clothing is worn before it is thrown away – decreased by 36%. This is an example of what is referred to by environmental economists as the Jevons paradox: increased efficiency leads to a rise rather than a decrease in consumption.
For energy efficiency to be impactful, then, it needs to be twinned with bigger changes to the economy.
The circular economy offers a framework to effect these changes. By eliminating waste and circulating products and materials through a combination of design and business model choices, the need to extract and process raw materials is significantly reduced, along with the associated energy needs. For example, where an organisation designs for and uses a remanufacturing business model, the use of secondary materials and reduced need for extraction, processing, and transport of natural resources could translate into energy savings in excess of 50%, according to the OECD.
The lower the demand for energy, the less renewable infrastructure we need to build, and the smaller the material impacts of this sector.
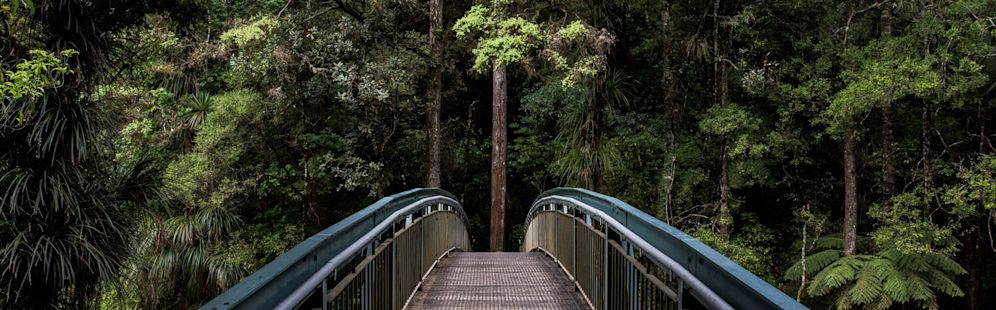
Room for more innovation
As with any complex industrial system, the transition from linear to circular in the renewable energy sector is no easy task. Experimentation, innovation, and collaboration between public and private actors is needed, along with policy and business changes.
There are a growing number of businesses and policymakers taking up the mantle. As Barry says: “I think we are approaching an inflection point born out of climate change, born out of a narrative that people are starting to get. I can list off countless examples of how we now see behaviours in business changing. We are in the business of culture change. This is about informing people that there is another way, there is a better way.”
Creativity is rife. Alongside circular design and new business models there are emerging innovations that could fundamentally reduce materials needs. This includes on-shore airborne wind energy that harnesses the potential of high-altitude winds while eliminating steel monopiles and long range wireless power transmission for offshore wind energy that eliminates the need for undersea cables. Though these are not silver bullets, they highlight to huge potential of this industry to not only produce cleaner energy but deliver it via infrastructure designed to eliminate waste and pollution, keep materials in use, and enable nature to thrive.
It’s also promising to see initiatives like the Global Alliance for Sustainable Energy emerging, which states, up front, its commitment “to face the sector’s environmental and social impact, also considering Human Rights concerns, caused by the widespread usage of raw materials in Renewable Energy equipment manufacturing.” It points directly to the need for a circular design approach to the renewable energy transition as being “the perfect enabler to solve this issue.”
Achieving net-zero by 2050 requires a global transition to renewable energy. This shift needs to be circular.
With thanks the following experts for their contributions
Anne Velenturf, Senior Research Fellow in Circular Economy, University of Leeds
Carmen Valache-Altinel, former Project Manager: Climate Change, Ellen MacArthur Foundation
Fiona Lindsay, Company Director, ReBlade
Giuseppe Della Greca, Circular Economy specialist, Enel Group
Ian Banks, former Editorial Lead, Ellen MacArthur Foundation
James Barry, CEO, Renewable Parts
Laura Collacott, Journalist and Communications Consultant
Lena Gravis, Senior Expert – Editorial, Ellen MacArthur Foundation
Matthew Chapman, Marketing Manager, Renewable Parts
Miranda Schnitger, Climate Lead, Ellen MacArthur Foundation
Nick Jeffries, Senior Expert – Insights and Analysis, Ellen MacArthur Foundation
Parikhit Sinha, Director, Sustainable Development/ Environmental, First Solar
Rebecca Windemer, ESRC Postdoctoral Fellow, Cardiff University
Roland Geyer, Professor in Industrial Ecology, Bren School of Environmental Science and Management, University of California at Santa Barbara
Ross Jaggers, former Programme Manager, Science Divisions, Research & Innovation, Royal Society of Chemistry (currently Insights Advisor, Xynteo)
Saar Bentein, Public Relations & Public Affairs Coordinator, Recupel
Scott Bryant, Circular Economy Coordinator, City of Greater Bendigo, and former Circular Energy Infrastructure Lead at Zero Waste Scotland
Tom Rommens, Coordinator, CIRCUSOL