By Tansy Robertson-Fall, Senior Editor, Ellen MacArthur Foundation
The renewable energyrenewable energyEnergy derived from resources that are not depleted on timescales relevant to the economy, i.e. not geological timescales. sector promises to tap into limitless sources of energy while tackling pollution and climate change. However, the materials needed to capture and store this energy are finite. As the industry scales at pace, renewable infrastructure designed within a ‘take-make-waste’ linear system could contribute to greenhouse gas emissions and biodiversity loss. To prevent this solution becoming a problem, renewable energy infrastructure needs to be built for a circular economycircular economyA systems solution framework that tackles global challenges like climate change, biodiversity loss, waste, and pollution. It is based on three principles, driven by design: eliminate waste and pollution, circulate products and materials (at their highest value), and regenerate nature..
"There's so much pressure and emphasis on getting the Green Revolution happening that it's almost by any means necessary without that pause of 'well it is green, but is it as green as it should be?"
- Scott Bryant, Circular Economy Coordinator, City of Greater Bendigo
It is unquestionable that renewable energy needs to be part of the solution to climate change. With many countries committing at COP26 to reach net-zero carbon emissions by 2050, renewable energy infrastructure is set to scale rapidly.
New onshore wind power capacity added in 2020 was twice that installed in 2019 and the US, Europe, China, and other countries have laid out plans to ramp up investments in renewables. These have been further accelerated by Russia’s recent invasion of Ukraine, with European Commission President Ursula von der Leyen stating, “The quicker we switch to renewables and hydrogen, combined with more energy efficiency, the quicker we will be truly independent and master our energy system.”
However, while vital to reach a net-zero ambition, the renewable energy industry is not without its challenges. In early 2020, images of diggers burying rows of decommissioned wind turbine blades against a picturesque mountain range in Casper, Wyoming, captured headlines. The coverage gave credence to renewable energy sceptics who question the environmental credentials of current renewable technologies, with detractors labelling the turbines "Not so green energy”.
While these critics are not without their own agendas, it is certainly true that as the wind industry grows this problem is set to extend – it is estimated there will be 43 million tonnes of wind turbine blade waste by 2050 – and the blades landfilled in Wyoming will not be the only example of waste from the renewable energy sector entering the environment. Lithium-ion battery waste is predicted to reach 2 million tonnes annually by 2030 and the International Renewable Energy Agency (IRENA) estimates that there will be up to 78 million tonnes of solar panel waste by 2050.
Data credits: Liu and Barlow, University of Cambridge | International Renewable Energy Agency
Expected solar panel and wind turbine blade waste by 2050
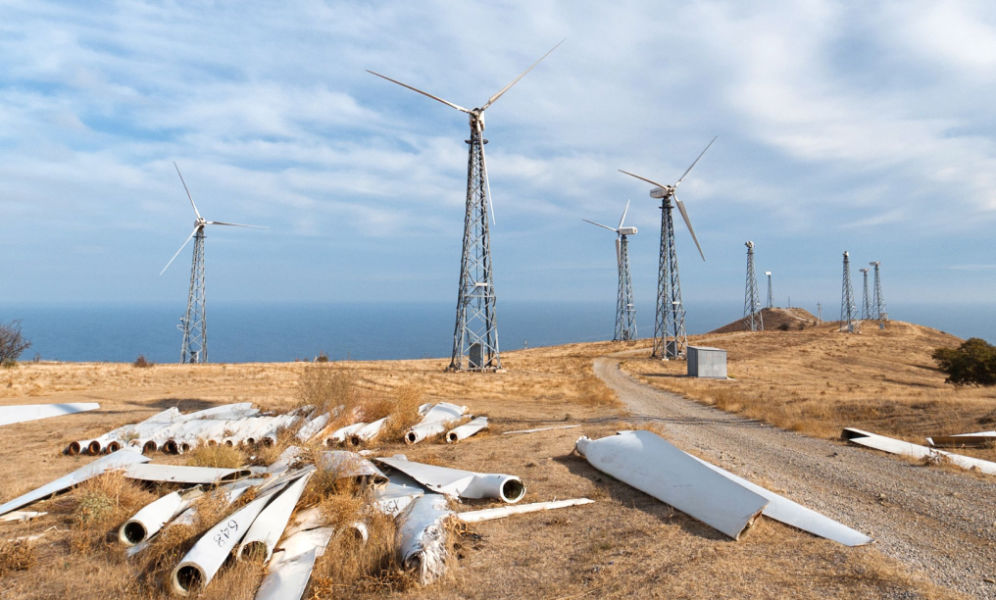
43 million tonnes of wind turbine blade waste
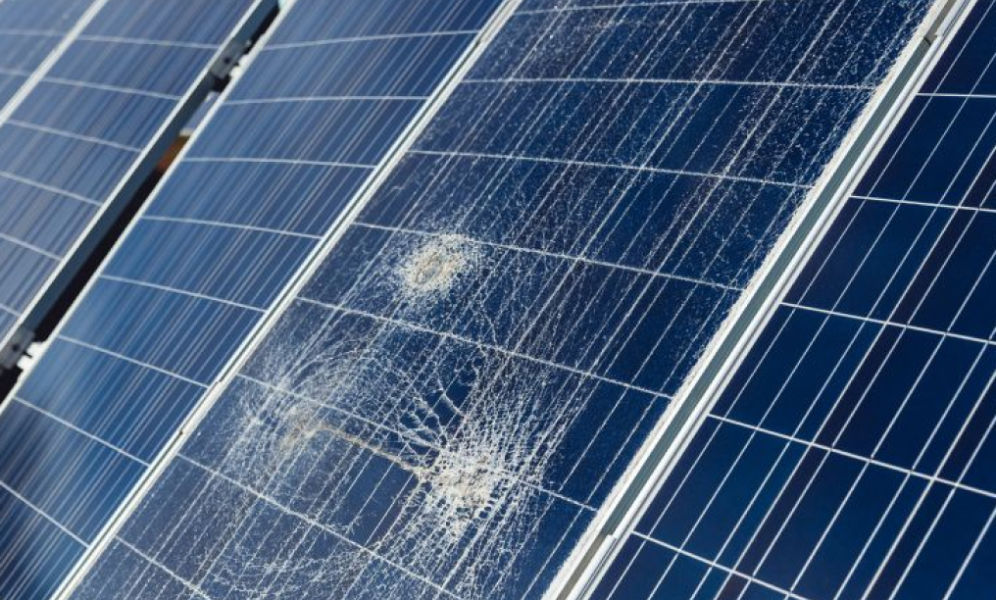
78 million tonnes of solar panel waste
This waste is currently an inevitability, the result of design and materials choices centred around two key factors: energy output and cost. These design choices have enabled renewables to compete with an established fossil-fuel industry.
The capacity of an individual wind turbine has more than doubled in the last 10 years, lowering the cost of wind energy, while developments in solar panel designs, along with policy support for the industry, have resulted in solar solutions that are “more cost effective than coal- and gas-fired power in many countries”, according to the International Energy Agency (IEA). For projects that have gained from low cost financing and that tap high quality resources, “solar PV is now the cheapest source of electricity in history”.
This is great news for the industry, and for nations looking to reduce their carbon emissions. But these same design choices have made it challenging and uneconomical to reusereuseThe repeated use of a product or component for its intended purpose without significant modification. and recyclerecycleTransform a product or component into its basic materials or substances and reprocessing them into new materials. components, including wind turbine blades made from low-value fibreglass composites, meaning they are destined for landfill or incinerators.
As James Barry, CEO of Renewable Parts – a business that has been endeavouring to keep large steel components from wind turbines in use and out of landfill since 2011 – comments, efforts to scale renewable infrastructure have been “to some extent, at the expense of looking at how the supply chain and the whole technology that sits behind it becomes more sustainable and greener. We are not necessarily a green supply chain.”
The impacts of this go far beyond waste.
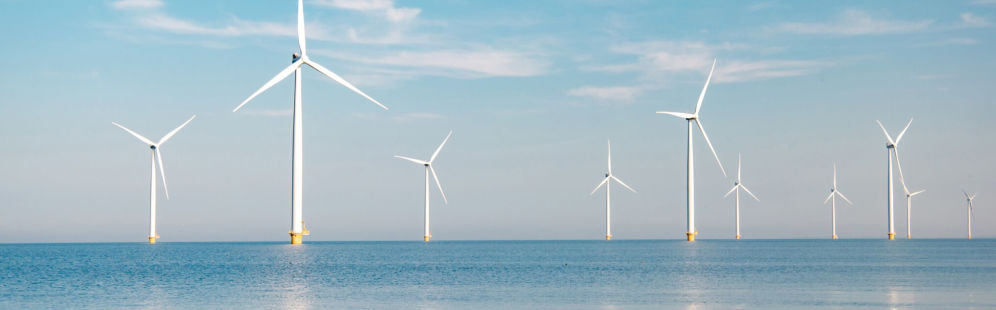
The current renewable energy supply chain has adverse impacts
The renewable energy sector is materials intensive. Each wind turbine, for example, is made from large quantities of steel, iron, fibreglass, copper, and aluminium (among other materials), erected on a concrete base. The potential negative impacts of these material needs as the industry scales are significant.
Take a 500 megawatt offshore wind farm like the forthcoming Sydkustens Vind development in the Baltic Sea, which will be capable of powering around 250,000 Swedish homes. A development of this size currently requires around 4,400 tonnes of copper.¹ Given that in 2050 it is estimated that installed wind power capacity will be 6 million megawatts, if half of this comes from offshore farms an estimated 27 million tonnes of copper will be needed to build the necessary infrastructure.
How much copper will be needed for offshore wind energy in the run-up to 2050?
How much copper will be needed for offshore wind energy in the run-up to 2050?
The growing appetite of the renewable energy sector for industrial materials could pit the industry against other sectors of the economy. It’s predicted that by 2050 demand for materials including steel, cement, aluminium, and plastics could quadruple across the economy, while mineral needs (including metals for renewable energy infrastructure) are expected to increase five times. This could lead to both price and supply concerns. Possible shortages mean prices are predicted to rise and remain elevated, spurred on by the shift to a carbon-neutral economy. Intensifying geopolitical and environmental challenges and constraints could also restrict availability as well as disrupting supply chains.
The US National Renewable Energy Laboratory (NREL) has highlighted risks associated with indium supply, for example, a key component in copper indium gallium selenide (CIGS) thin-film solar cells. Indium is most often sourced as a byproduct of zinc production and the NREL notes the benefits of sharingsharingThe use of a product by multiple users. It is a practice that retains the highest value of a product by extending its use period. production costs with this associated product. If indium demand increases and exceeds the amount available as a byproduct, the cost of producing indium will also rise and availability may come into question, making sourcing more challenging and constraining growth of CIGS technology.
In addition to these challenges, extraction and production of key industrial materials and components has significant environmental, and related societal, impacts. Cement manufacturing alone produces 2.2 billion tonnes of CO2 every year – 8% of global CO2 emissions. Meanwhile, for every tonne of copper mined, more than a tonne of CO2e is emitted.
Materials used in smaller quantities in renewable energy technologies can have equally negative impacts. A wind turbine’s generator – the nacelle – for example, is filled with rare earth metals such as neodymium and dysprosium. An MIT study estimates that a 2 megawatt wind turbine contains a little more than 340 kilograms of rare earths. While this is a relatively small proportion of the wind turbine’s weight, the processes used to extract rare earths are environmentally harmful, being achieved through chemical-intensive processes that pollute the air, soil, and groundwater. Extraction of materials such as lithium and cobalt for batteries, and silicon and zinc for solar panels also have negative environmental repercussions.
Mining for these materials threatens global biodiversity. While mining is widely accepted to have negative consequences for the environment – including local species extinction and environmental damage up to 20 times the area leased for the mines themselves – these impacts could be particularly acute for materials needed for renewable energy technologies. A paper published in Nature Communications found that “mining threats to biodiversity will increase as more mines target materials for renewable energy production and, without strategic planning, these new threats to biodiversity may surpass those averted by climate change mitigation.”
"Having an electric car doesn't mean you're driving without impact. It still has a considerable environmental impact."
- Roland Geyer, Professor in Industrial Ecology, Bren School of Environmental Science and Management, University of California at Santa Barbara
The social implications, particularly in resource-rich developing countries, of mining for the materials needed to build renewable technologies also shouldn’t be underestimated. The Institute for Sustainable Futures notes “health impacts on workers and surrounding communities”. In January 2021, the Supreme Court of Chile, the world’s biggest producer of copper, suspended operations at the Cerro Colorado copper mine following complaints by local indigenous communities about the project’s water use and the threat it poses to livelihoods and local wetlands.
The international effort to address climate change through a necessary transition to renewable energy is therefore not without environmental and social impacts. However, the renewable energy transition can be designed in such a way that it tackles not only climate change but also contributes to the mitigation of biodiversity loss and helps address global inequalities.
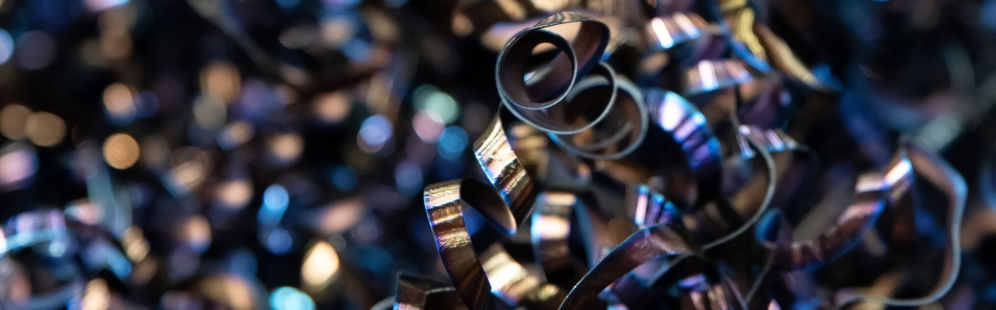
Sourcing materials from within the economy: a good place to start
Building the renewable energy sector in a way that minimises extraction of finite resources, limits business risks, and reduces negative climate, biodiversity, and social impacts necessitates using materials that have already been used. This is one of the core principles of a circular economy.
Large quantities of materials that could be reused systematically become waste. In 2020, approaching 800,000 tonnes of copper was disposed of in the form of batteries, electrical and electronic equipment, and vehicles in the EU, Norway, Switzerland, and the UK alone. This is only a small proportion of the copper that’s estimated to be unused and wasted globally.
Under the city of Norrköping, Sweden, which covers less than 36 square kilometres, it is estimated there are 508 tonnes of copper in old unused cables. If similar quantities of copper were under urban land across the European Union, and even if only half were available for use, that would amount to more than 3 million tonnes of copper. That’s enough to build more than 780 offshore wind farms the size of Sydkustens Vind and power all of the homes in the EU.
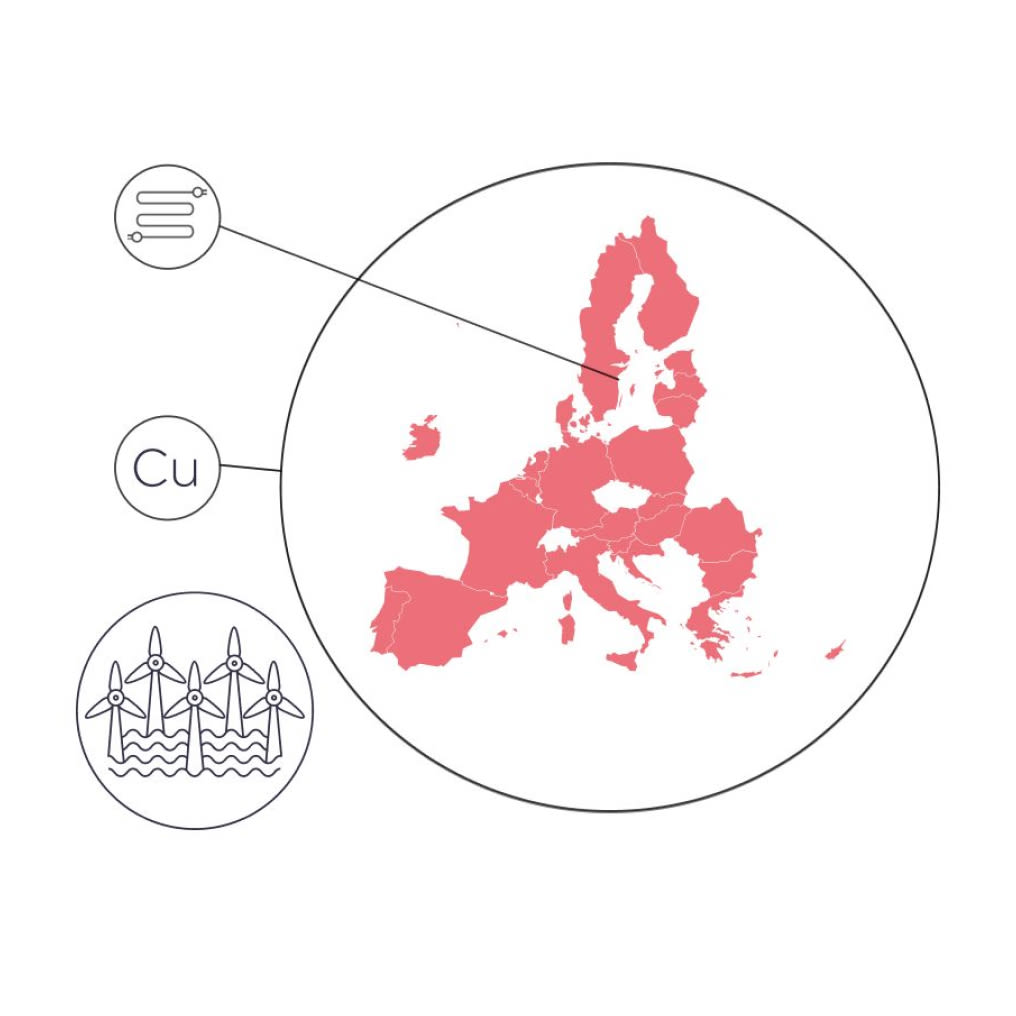
Potential secondary copper stocks under urban land in Europe
508 tonnes of copper have been found in unused cables under Norrköping, Sweden. If similar quantities of copper were under urban land across the European Union, and even if only half were available for use, that would amount to more than 3 million tonnes of copper – enough to build more than 780 offshore wind farms the size of Sydkustens Vind and power all of the homes in the EU.
Reusing materials to build renewable energy infrastructure could also offer a financial opportunity for some businesses. Major oil companies, such as Royal Dutch Shell, Total, BP, Eni, and Equinor, are investing in renewable energy infrastructure – as pressure mounts to phase out fossil fuels – while also gearing up to absorb their share of fossil-fuel decommissioning costs, projected to be USD 42 billion by 2024. There is potential to offset some costs in both areas by reusing existing fossil-fuel infrastructure to build renewable technologies.
In 2019, Eni installed its first wave turbine (formally an Inertial Sea Wave Energy Converter) that it states “in the future, will enable Eni to convert mature offshore platforms into renewable energy generation hubs.” Where conversion isn’t possible, oil rig decommissioning could see vast quantities of secondary steel become available – UK owned oil rigs alone held more than 4.6 million tonnes of steel by 2013 – which could be recycled for use in wind turbine monopiles and solar panel mounts.
Building the secondary materials market requires actions by policymakers, often with business buy-in. Legislation like the WEEE directive, which aims to ensure electrical and electronic equipment in Europe does not become waste, is beginning to feed such a market and the introduction of recovery streams like this around the world could play an important role in the development of renewable energy infrastructure. Recovering materials from discarded mobile phones, for example, could provide a source of valuable metals including indium, a vital component of many solar panels. Harnessing these existing materials – so-called ‘urban mining’ – could significantly reduce extraction- and production-related greenhouse gas emissions.
While only 8.5 million tonnes of copper is recycled annually, by using copper scrap CO2 emissions can be reduced by 65% and current global copper recycling efforts reduce CO2 emissions by 40 million tonnes annually, equivalent to taking 16 million cars off the road. Similarly, using recycled steel, which is responsible for 50% of the carbon footprint of a wind turbine, could reduce CO2 emissions by around 60% compared to the production of steel using raw materials.
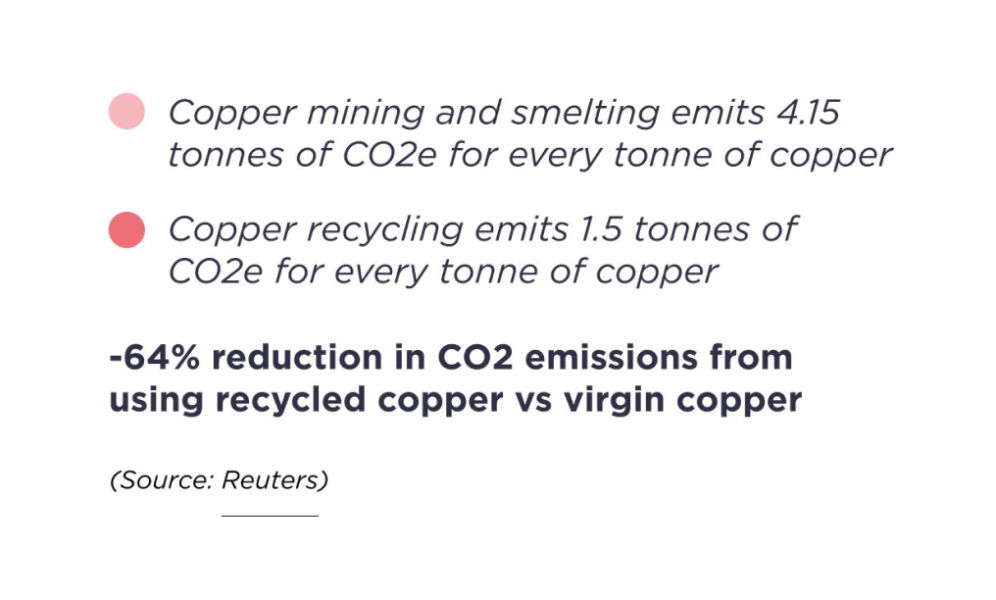
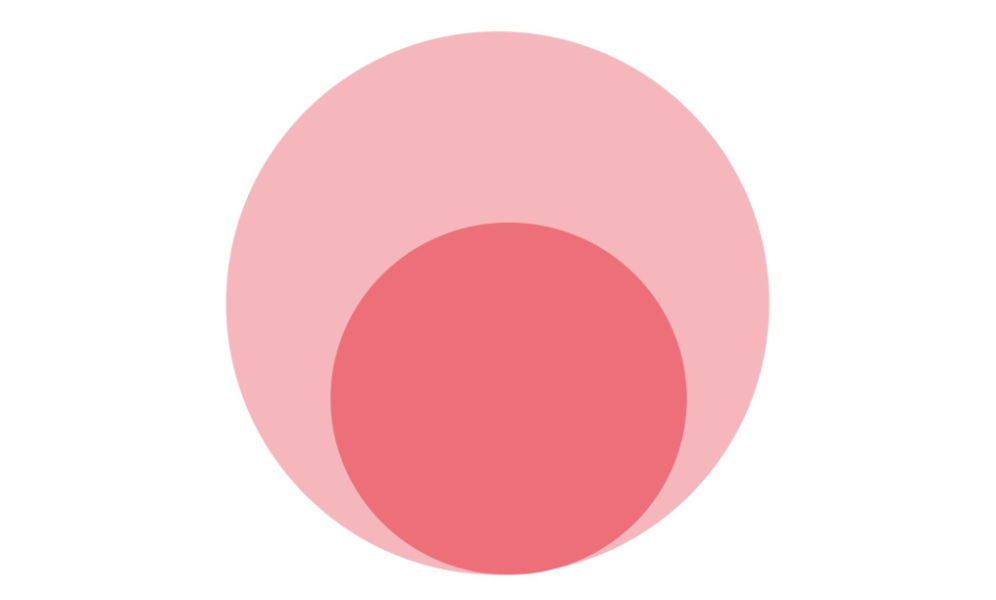
Use of secondary materials could equally prevent extractive mining in areas rich in biodiversity – notably in countries such as Brazil and Australia, which together are home to more than 1800 endemic species of mammal, birds, and amphibians but also undertake extensive mining operations. To achieve the forthcoming biodiversity target – declared at the recent COP in Kungming – to ‘protect and conserve 30% of land and sea areas’ by 2030, such prevention will be necessary.
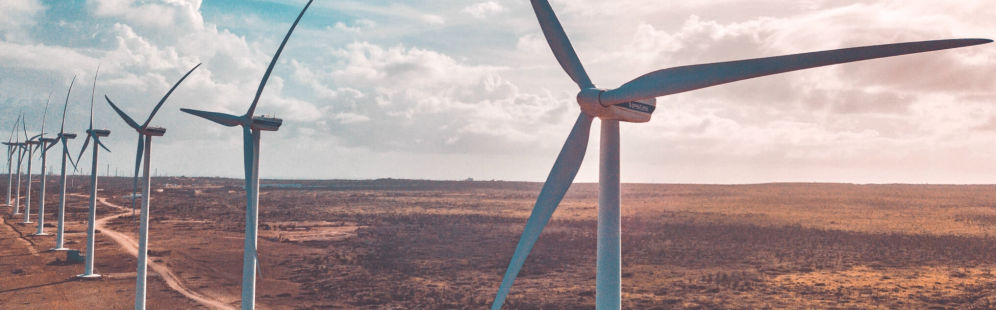
The renewable energy industry needs to embed a circular economy at the design stage
While much needed, these urban mining activities are framed by the current take-make-waste paradigm of the linear economylinear economyAn economy in which finite resources are extracted to make products that are used - generally not to their full potential - and then thrown away ('take-make-waste')., in which each sector inevitably produces waste. Looking to harvest this waste can act as a short term solution to scale the renewable energy industry but for it to be carbon-neutral long-term, renewable energy infrastructure needs to be designed for a circular economy in which waste and pollution are eliminated, materials are circulated, and nature is regenerated.
This means fundamentally rethinking the architecture of renewable energy infrastructure, the policy mechanisms that inform use-lifetimes and end of use actions, as well as the business models that the industry is built around to avoid the creation of waste and significantly reduce the need for virgin materialsvirgin materialsMaterials that have not yet been used in the economy. needs and the negative climate, biodiversity, and societal impacts of their extraction and processing. The principles of the circular economy offer a future-proofed way of mitigating climate change.
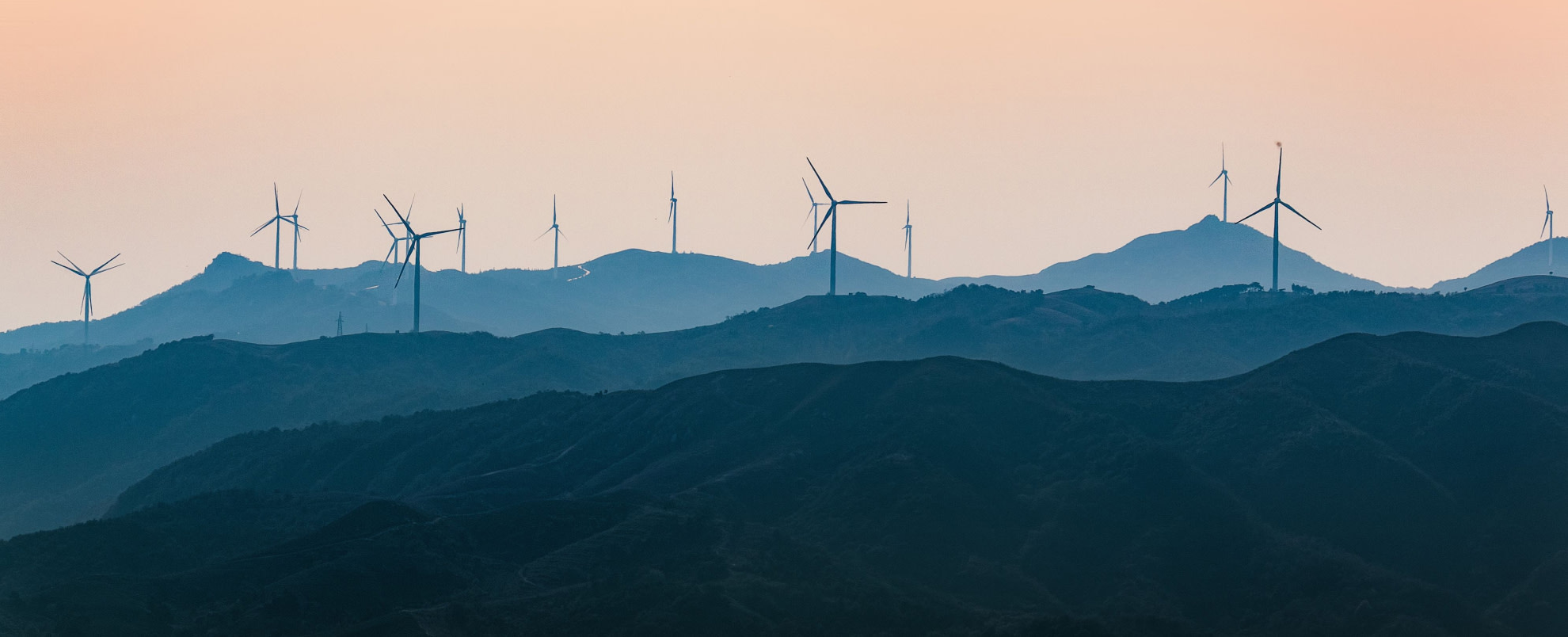
Continue reading with part 2
We need to talk about renewables is a two-part article series.
With thanks to the following experts for their contributions
Anne Velenturf, Research Impact Fellow in Circular Economy, University of Leeds
Carmen Valache-Altinel, former Project Manager: Climate Change, Ellen MacArthur Foundation
Ian Banks, Editorial Lead, Ellen MacArthur Foundation
James Barry, CEO, Renewable Parts
Laura Collacott, Journalist and Communications Consultant
Lena Gravis, Senior Expert – Editorial, Ellen MacArthur Foundation
Matthew Chapman, Marketing Manager, Renewable Parts
Nick Jeffries, Senior Expert – Insights and Analysis, Ellen MacArthur Foundation
Parikhit Sinha, Director, Sustainable Development/ Environmental, First Solar
Rebecca Windemer, ESRC Postdoctoral Fellow, Cardiff University
Roland Geyer, Professor in Industrial Ecology, Bren School of Environmental Science and Management, University of California at Santa Barbara
Ross Jaggers, former Programme Manager, Science Divisions, Research & Innovation, Royal Society of Chemistry (currently Insights Advisor, Xynteo)
Saar Bentein, Public Relations & Public Affairs Coordinator, Recupel
Scott Bryant, Circular Economy Coordinator, City of Greater Bendigo
Tom Rommens, Coordinator, CIRCUSOL
Footnote: ¹ Rounded average. Sources: Copper Development Association (2019); IEA (2021).